Dossier
Photovoltaics on agricultural land – for a rapid energy turnaround
Folkhard Isermeyer | 28.11.2022
Germany has enough land to supply itself completely with renewable energy. The combination of wind energy and ground-mounted photovoltaics is optimal for a rapid energy turnaround. This does not jeopardise the security of the food supply. However, the expansion concepts for ground-mounted PV should be reversible.
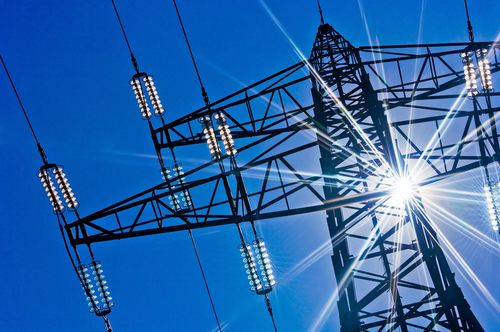
When it comes to energy supply, the theory that self-sufficiency in energy is unattainable for Germany persists. Therefore, in the post-fossil age, our country would have to import a considerable part of the solar and wind power, be it as electricity via high-voltage lines, or as gas or liquid in tankers or pipelines.
This hypothesis is now outdated. In particular, the enormous technical advances in photovoltaic systems will open up the possibility for Germany to become completely self-sufficient in renewable energy within the next two to three decades. Whether self-sufficiency is actually implemented or whether Germany ultimately decides to import part of its energy needs is therefore not a question of physical necessity ("we have to import"), but a question of competitiveness and political volition ("we can import").
Energy transition: "Business as usual" does not lead to the goal
Figure 1 shows how energy consumption in the three major segments has developed over the past 30 years and what shares renewable energies have achieved [1]. At first glance, it is clear that if we continue on as we have been doing, i.e., if we continue the previous trends simply continuing with this policy, we will not even come close to achieving the targeted climate neutrality of the national economy by the middle of the century - nor will we be independent from energy imports.
However, there is an opportunity to flip the proverbial switch. Significant impulses are being provided by the electrification of the heating/cooling and transport segments. With the help of heat pumps, 2.6 to 3.1 kWh of heat can be generated from one kilowatt hour (kWh) of electricity under practical conditions if ambient heat is used [2] [3]. The transport sector can also be made more efficient, at least in the passenger car sector: Electric motors convert significantly more of the energy used into motion, while in internal combustion engines a large part of the energy supplied escapes as useless heat.
These transformations will bring considerable efficiency gains. At the same time, energy supply is focusing more and more on electricity. The demand for electricity is rising sharply.
Hydrogen or methane instead of electricity?
At present, no one can predict exactly which parts of the heating/cooling and transport segments will be electrified and which parts will be supplied by hydrogen or methane in the long term. [4] [5]
This situation can be ignored at the moment. The decisive factor is that the main source of hydrogen and methane in the post-fossil, climate-neutral age will also be regeneratively generated electricity. The more the decisively the "diversions" via green hydrogen are taken, the greater the overall electricity demand will be, because each conversion step causes energy losses, some of which are considerable.
How much electricity will be required at the end? This depends on many assumptions. Estimates for the mid 21st century span a wide range of uncertainty, ranging from about 580 terawatt hours (TWh) per year [6] [7] at the lower end, to 2,400 TWh/year [8] at the upper end. The projections at the lower end assume only partial electrification of the energy economy and continue to include imports of energy sources.
Since the following calculations are made for the (extreme) scenario of complete self-sufficiency for Germany, we conservatively assume an electricity demand of 2,000 TWh/year.
Where will the electricity come from?
Solar and wind energy are by far the most promising candidates for a self-sufficient power supply, not least because of the great technological advances. According to calculations by the Fraunhofer Institute for Solar Energy Systems (ISE) [9], ground-mounted photovoltaics (PV) and wind energy provide the most cost-effective electricity (Fig. 2). The cost values shown in the figure reflect the 2021 price ratios, i.e., they do not yet include the special prices and interest rate increases of 2022.
Compared to energy generation from biogenic sources (wood from sustainable forestry, energy crops on arable land), the advantage of solar and wind energy lies not only in the lower costs, but also in the significantly higher yields per hectare. The following orders of magnitude apply here (megawatt hours per hectare):
- Bioenergy: 10 to 60 MWh/ha1
- Open space PV: 1,000 MWh/ha2
- Wind energy: 20,000 MWh/ha3
What happens when there is no sun and no wind?
The great challenge of an energy transition based primarily on wind and solar energy is energy storage. [14] [16] [17] [18] In the case of solar and wind power, temporary oversupply and undersupply occur both during the course of the year and during the course of the day, this must be balanced out.
To balance out over- and undersupply over the course of the year, it is, in principle, favourable for wind and solar energy to be expanded roughly in step with each other. Both seasonal curves complement each other well (cf. Fig. 3) [19]. Nevertheless, the supply system must be able to compensate for windless phases of several weeks in the winter half-year (so-called "dark lull"). For this purpose, an infrastructure must be created that (a) converts surplus electricity into hydrogen with electrolysers, (b) stores this hydrogen in caverns and (c) "reconverts" the hydrogen into electricity in times of deficit.
Technical and organisational solutions must also be created to balance out the over- and under-supply during the course of the day. It is expected that large-scale PV home and battery storage systems on the order of 100 and 60 GW respectively will be needed for this [20]. In addition, the supra-regional (European) grid interconnection, as well as the development of intelligent load management, including weather forecasts, are of importance here. In the energy world of tomorrow, people and end devices (charging stations, washing machines, etc.) will permanently receive price signals that show them at short notice when they can use cheap electricity or when it is better not to use expensive electricity. Many millions of people and devices will then contribute flexibly and cost-effectively to matching demand with supply without having to resort to the relatively expensive reconversion of electricity.
Creating the necessary infrastructure will take two to three decades. However, the expansion of solar and wind energy does not have to wait for this infrastructure. Rather, this expansion can and must take place in parallel, so that the energy transition is completed by the middle of the century.
How much land is needed for energy self-sufficiency?
If wind and solar energy are expanded in step, in the long-term perspective each of these two sources would have to supply a maximum of 1,000 TWh per year.
For wind energy, the following rough calculations illustrate the resulting land requirements: Two thirds of the wind energy will probably be generated onshore, one third offshore. For each onshore wind turbine, about one third of a hectare will be sealed and (after repowering of the old turbines) 6,000 to 10,000 MWh/year will be harvested. A total of around 70,000 to 100,000 turbines will then be needed. The land required for this is 20,000 to 30,000 hectares. With an estimated 25,000 to 35,000 villages in Germany [21] and an assumed equal distribution of wind turbines throughout Germany, this would result in a turbine density of two to three per village and four square kilometres per turbine.
For photovoltaics, we initially assume in an extreme scenario the complete generation of 1,000 TWh/year as open space PV on existing agricultural land. For this scenario, a land requirement of one million hectares is calculated with a yield of 1,000 MWh/ha. However, this value applies to an extreme scenario. In reality, it can be assumed that even in the near future a considerable part of the PV electricity will be generated on already sealed surfaces (roofs, car parks, etc.) and thus much less agricultural land will be needed. Estimates based on current political goals and various energy scenarios come to a probable land use of 280,000 hectares for the year 2040 [22].
Does open space PV endanger our food supply?
Currently, 2.3 million hectares are used for the cultivation of energy crops in Germany [23]. The production of biodiesel and bioethanol also generates by-products for animal feed, so that the "net land use" for bioenergy is just under 2 million hectares. This land is no longer needed in the solar/wind scenario. The gradual switch from energy crop production to photovoltaics will therefore not worsen the food supply, but on the contrary significantly improve it.
However, this does not at all mean that we are moving towards a relaxed future without land competition. Other land demands are growing. Germany's chemical industry currently uses about 18 million tonnes of fossil raw materials and only just under 3 million tonnes of renewable raw materials [24]. In the post-fossil age, a large part of the carbon carriers for organic chemistry will be obtained from agriculture and forestry. Depending on the raw material mix, up to five million hectares may be required for this. Further land requirements arise from the fact that we need additional land to achieve climate protection and biodiversity goals. In addition, about 40,000 hectares of land are lost for agriculture every year due to land conversion [25]. Thus, there are many reasons to be very careful with scarce agricultural land in the future.
The greatest leverage lies in reducing livestock farming. Currently, 60 per cent of agricultural land is used for animal feed production. A lot of energy is consumed in the production of meat: When we eat meat, the energy yield per hectare is on the order of three to six MWh/ha, whereas with a plant-based diet (e.g., wheat/soya) it is around 20 MWh/ha (see above, calculations based on LfL data). Therefore, there is much to be said for orienting animal husbandry more towards the utilisation of permanent grassland, using arable land more for the production of plant-based food for humans and reducing meat consumption. Policymakers could support this, as has often been called for, through a VAT reform by lowering the tax rate on plant-based food to zero and raising the tax rate on animal-based food to 19 %.
Can the energy transition succeed without ground-mounted PV?
The solar/wind combination is not necessarily dependent on domestic agricultural land being used for ground-mounted PV, because there are alternatives.
Within Germany, there are numerous possibilities for integrating PV systems into the residential/transport sector (roof surfaces, facades, car parks, etc.). In this way, a potential of more than 1,000 TWh/year can be tapped in the long term [14]. However, many adaptation measures are currently still relatively expensive. In part, they are also hindered by planning law. In the course of the next few years and decades, however, it will become increasingly possible to tap this potential.
The second alternative is Agri-PV, i.e. the integration of PV systems into agricultural production systems. The charm of this option lies in the fact that food continues to be produced on agricultural land. Even if the yield level is somewhat reduced, a kind of double dividend is still achieved. However, these advantages are often offset by considerable additional costs. Since most of the concepts are currently still at the experimental stage, a rapid broad-scale effect is at the moment not available - unless the concepts are artificially lifted into competitiveness by the EEG subsidy.
The third alternative is the import of solar or wind power from sunnier or windier parts of the world. The import can take place as electricity import (via high-voltage direct current transmission) or as material import of gaseous or liquid energy sources (via ship or pipeline). The production costs in those parts of the world are even lower than here because there are many hours of sunshine and because solar thermal energy, photovoltaics and energy storage in salt tanks can be combined [26] [27]. However, additional investment costs for the construction of power lines (high-voltage direct current transmission) as well as transport losses on the order of 14 % must be taken into account [14]. Whether the import of renewable energy will ultimately reach significant levels will be determined by competition over time. In the next two decades, however, we can hardly expect any significant electricity imports, if only because the producing countries will initially use a large part of the energy generated from renewable sources to cover their own needs.
Conclusion: In the distant future, Germany will be able to generate sufficient renewable energy even without ground-mounted PV. However, if our society wants to achieve a fast and cost-effective energy transition, ground-mounted PV is the best option for this.
How much can bioenergy support the energy transition?
So far, the majority of renewable energy has been provided by bioenergy (cf. Figure 1), mainly through the three components (a) wood combustion, (b) biogas based on maize and (c) bio-diesel based on rapeseed.
If Germany wants to be climate neutral by mid-century, and predominantly self-sufficient in energy and food, this goal cannot be achieved with bioenergy. Our agricultural land is expected to be only 15 million hectares in the second half of the century. However, the production of 1,000 TWh/year would require more than 20 million hectares of bioenergy land. This would make self-sufficiency in food or the greening of agricultural production unthinkable.
In this respect, it makes sense to focus consistently on wind and solar in strategic planning now. The land that is currently used for the cultivation of energy crops can then gradually be freed up for other uses. How quickly this process is completed is a question of political trade-offs. Currently, both energy and food prices are at very high levels. If this exceptional scarcity continues for a few more years, policymakers may consider not restricting bioenergy production for the time being, but rather imposing greater restrictions on consumption and animal food production (see above: VAT reform).
If the cultivation of energy crops is then ended in the longer term, this by no means means the end of bioenergy. The agricultural, food, forestry and timber industries produce many residual and waste materials that can be used for energy. The extent to which this is done depends on the eco-efficiency of the various use chains and will ultimately be decided by competition.
The recommendation to also phase out wood burning in the long term is justified by climate policy. If we do not harvest mature trees but leave them in the forest, they initially continue to store carbon there. However, the forest then grows towards its climax stage and can, after it has reached this saturation stage, no more additional carbon can be sequestered. It therefore makes more sense for climate protection if we harvest the trees, use the wood in the construction sector and store it permanently in dry conditions, for example in mines. As long as we don't burn the wood or let it rot, we can keep increasing the carbon storage. Then the used forest acts like an "air filter" with which we can recapture the CO2 that we have released by burning fossil fuels in the past in the centuries to come.
Conclusion and outlook
The following conclusions can be drawn from the analysis:
- If we consistently focus on wind and solar energy in the energy turnaround, we will have sufficient opportunities to cover Germany's entire energy needs completely from domestic generation in the long term.
- For a fast and cost-effective energy transition, it makes sense to allow and promote a vigorous expansion of ground-mounted PV in the coming years in addition to wind energy. However, this process should be reversible, as other PV concepts may gain preference over time.
- The security of food supply is not endangered by the expansion of ground-mounted PV. On the contrary: the gradual replacement of bioenergy with solar energy means that much more renewable energy can be produced overall, but less land is needed for this than before.
Under the current price conditions, which are, however, massively influenced by the consequences of Russia's aggression policy, the expansion of wind and solar energy is highly profitable. As long as prices do not change fundamentally, the expansion would take place step-by-step by itself, without the need for state support - at least for this part of the energy transition.
However, from the perspective of energy producers, who still have the ups and downs of the past two decades in their bones, the outlook is much more cautious. Price conditions on the international markets can change abruptly, supply chains can be interrupted, licensing procedures can put the brakes on entrepreneurial initiatives, and changes in energy and climate policies can make business models unprofitable. In addition, there is resistance among farmers, especially in small-scale agricultural regions. Many of them are concerned that some investors and landowners will share the economic returns of the PV boom among themselves, while most farmers in the region will go empty-handed and gradually lose their basis of production. From their point of view, there is no transparent dialogue about the expansion of renewable energies in their region, and they miss business models with which they can profit from the returns of the energy transition. These multiple uncertainties stand in the way of a dynamic energy transition.
It is therefore the task of politicy-makers to give the desired transformation a clear orientation and to optimise the legal and funding framework.
Of paramount importance for the entire energy transition are two tasks that fall under the responsibility of the Federal Ministry of Economics and Climate Protection (BMWK) at the federal level:
- Rapid establishment of an efficient industry for the production of solar systems (not necessarily in Germany, but predominantly in the EU to secure energy sovereignty),
- rapid expansion of the grid and storage infrastructure (in Germany).
In addition, there are also important tasks at the interface of land use and energy policy that require targeted cooperation between the federal ministries of agriculture, of the environment, of economic affairs and climate protection, as well as with the federal states:
Agreement on the targeted expansion path for ground-mounted PV (for Germany as a whole, but also for the spatial distribution of ground-mounted PV),
- Further development of the electricity market design (objectives: ensure continuous growth even with strong market fluctuations, develop an efficient supply system, ensure a fair balance of interests),
- Further development of approval procedures (better integration of planning for wind and PV at the regional level; increased citizen participation in detailed planning at the municipal level; assistance for the lower planning authorities),
- Promotion of supra-regional structures for best-practice sharing between existing and planned solar parks (also with regard to nature conservation, biodiversity, landscape, economic co-use),
- Agreement on time paths and concepts for the gradual phase-out of energy crop cultivation and wood combustion (phase-out is not necessary in the short term; however, long-term time paths and concepts are needed for stakeholders' planning).
In research policy, energy storage concepts undoubtedly deserve the highest priority. However, there are also important tasks at the interface between land use and energy production. For example, the federal and state governments could jointly develop a powerful research concept for agri-PV, also in order to better network the initiatives that are underway in many places.
- Federal Ministry for Economic Affairs and Climate Protection (2022): Facts and figures. Energy data.
- Bergmann A, Erhorn H (2017): Energy efficiency of electrically driven heat pumps. Results from monitoring. Fraunhofer Institute for Building Physics, IBP Communication 549.
- Günther D, Wapler J, Langner R, Helmling S, Miara M, Fischer D, Zimmermann D, Wolf T, Wille-Hausmann B (2020) WPsmart im Bestand: Felduntersuchung optimal abgestimmter Wärmepumpenheizungssysteme in Bestandsgebäuden beim Betrieb im konventionellen sowie im intelligenten Stromnetz (Smart Grid), ed. v. Fraunhofer Institute for Solar Energy Systems ISE (Fraunhofer ISE), https://www.ise.fraunhofer.de/de/forschungsprojekte/wpsmart-im-bestand.html [cited 12.9.2022].
- Clausen J (2022): The hydrogen dilemma. Availability, needs and myths. Berlin, Borderstep Institute
- Kreidelmeier S, Damecken H, Kirchner A, Wünsch M (2020): Costs and transformation pathways for electricity-based energy sources. Prognos.
- Purr K, Günther J, Lehmann H, Nuss P (2019) Pathways to resource-efficient greenhouse gas neutrality: RESCUE - Study, ed. by Federal Environment Agency (UBA), 444 p, found in https://www.umweltbundesamt.de/rescue [cited 3.11.2020].
- Prognos, Fraunhofer ISE [Fraunhofer Institute for Solar Energy Systems ISE], GWS, iinas (2020) Energiewirtschaftliche Projektionen und Folgeabschätzungen 2030/2050: Dokumentation von Referenzszenario und Szenario mit Klimaschutzprogramm 2030, ed. by Federal Ministry for Economic Affairs and Energy (BMWi), 104 p, to be found in https://www.bmwi.de/Redaktion/DE/Publikationen/Wirtschaft/klimagutachten.pdf? blob=publicationFile& v=8 [cited 20.10.2020].
- Fraunhofer Institute for Energy Economics and Energy System Technology (2019): The barometer of the energy transition. Kassel
- Kost C, Shammugam S, Fluri V, Peper D, Memar AD, Schlegel T (2021): LCOE of renewable energies. Fraunhofer Institute for Solar Energy Systems ISE
- Bayerische Landesanstalt für Landwirtschaft (2022): LfL contribution margins and calculation data. On the Internet at https://www.lfl.bayern.de/iba/unternehmensfuehrung/088966/index.php
- Agency for Renewable Resources (2022): https://biogas.fnr.de/daten-und-fakten/faustzahlen/
- Schober R (1987): Yield tables of important tree species. J. D. Sauerländer's Verlag, Frankfurt/Main
- Scientific Advisory Council on Agricultural Policy at the BMELV (2007): Use of biomass for energy production. Berlin
- Wirth H (2022): Current facts on photovoltaics in Germany. Fraunhofer Institute for Solar Energy Systems ISE, version of 17.07.2022.
- Südwerk (2022): https://www.s-werk.com/projekte/
- German Energy Agency (2020): dena study System Security 2050
- Agora Energiewende (2014): Stromspeicher in der Energiewende.
- Wirth H, Kost C, KramerK, Neuhaus H, Peper D, Rentsch J, Senkpiel C (2021): Solar offensive - How we unleash an economic boom and protect the climate with solar energy. Fraunhofer Institute for Solar Energy Systems ISE.
- Fraunhofer Institute for Solar Energy Systems ISE (2022): https://www.energy- charts.info/downloads/Power_generation_2021.pdf
- 50Hertz Transmission, Aprion, TenneT TSO, TransnetBW (2022): Scenario framework for the electricity network development plan 2037 with outlook 2045, version 2023: Draft by the Transmission System Operators, 125 www.netzentwicklungsplan.de/sites/default/files/paragraphs-files/Draft_NEP2037_2023.pdf [cited 18.1.2022].
- Steinführer A (2021): Can the number of villages in Germany be determined? Reports on Agriculture, Vol. 99, Issue 3
- Böhm J, Tietz, A. (2022): Estimation of the future area requirements of ground-mounted photovoltaic systems,
Thünen Working Paper 204. DOI:10.3220/WP1669630417000
- Fachagentur Nachwachsende Rohstoffe e.V. (2022): Basic Data Bioenergy Germany 2022
- Verband der Chemischen Industrie e.V. (2019): Raw material base of the chemical industry. Facts and figures.
- Federal Statistical Office (2022): Land area (actual use)
- Pitz-Paal R (2019): Controllable solar power - soon to be competitively cheap for the first time in North Africa. On the Internet at www.dlr.de/content/de/artikel/news/2019/02/20190605_regelbarer- solarstrom_in-nordafrika- erstmals-konkurrenzfaehig-guenstig.html
- Agora-Verkehrswende, Agora-Energiewende and Frontier Economics (2018): The future costs of electricity- based synthetic fuels
The text goes back to a lecture given by the author on 8 November 2022 at the Strategic Forum "Land Use in Transition" held by the DAFA. This article can be found on the Thünen website in the topic area "Long-term policy concepts".